Revisited: Your Guide to SALINITY
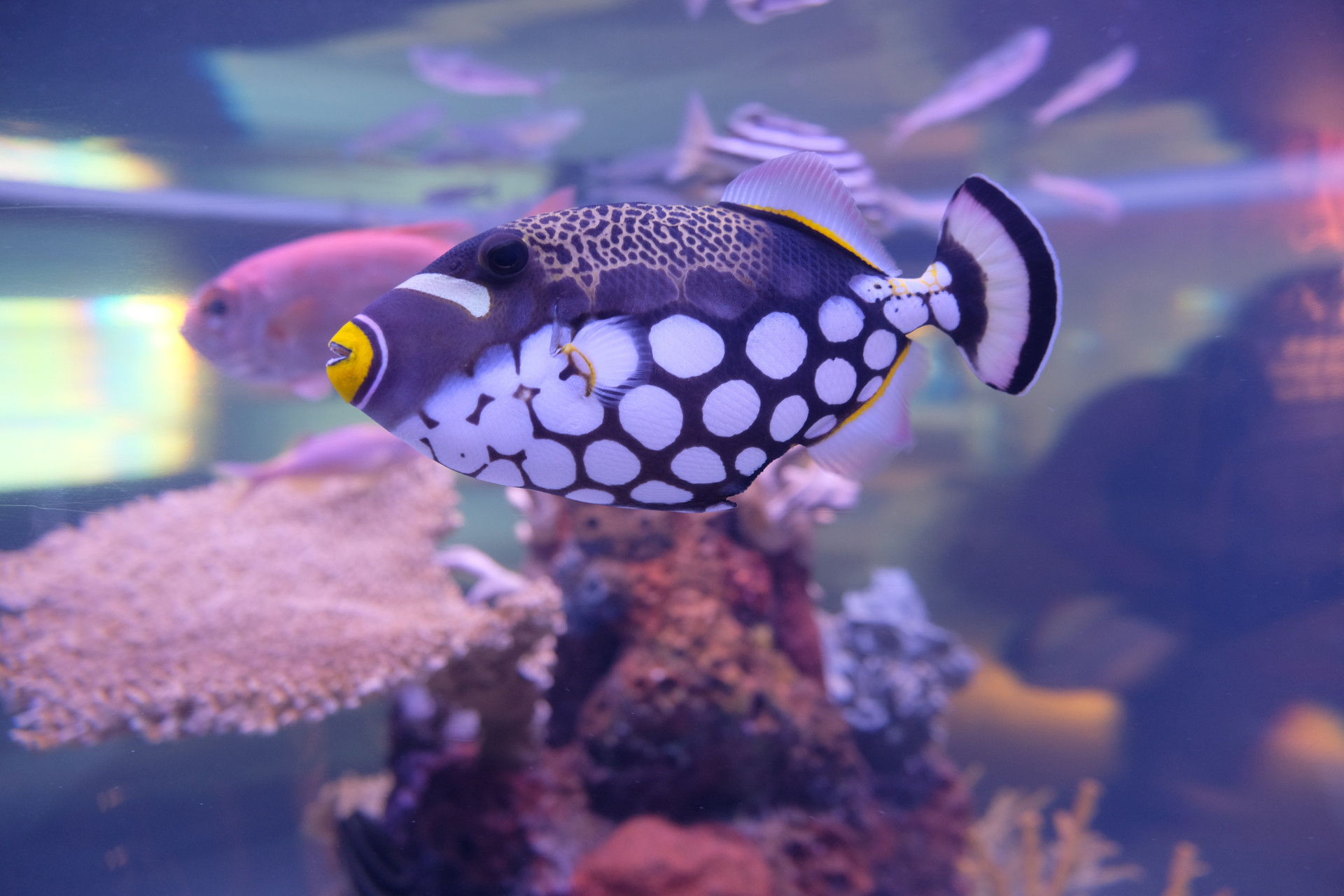
Preface
It is time for yet another edition of Chemistry Corner, and today the topic in question is salt, or rather, salinity. As a marine aquarist, you no doubt understand that salinity levels have an effect on the health of the livestock in your tank. You probably understand that there is desirable range within which saltwater tanks must reside, or else they perish. At the same time, it has always seemed to me that salinity gets only a cursory explanation. It always goes something like salinity should have a specific gravity ranging from 1.021 to 1.025, and natural seawater has a salinity of 1.024. What does that really mean though, and why is that the range? And what happens if salinity falls outside those end points?
Why Do We Have a Salty Ocean?
To better understand the role of salinity in the marine environment, we must first gain comprehension of the sources of oceanic salt. In the beginning, when the primordial Earth was a hellish cauldron of volcanism, lava, and scorching gases, there was no liquid water. Instead, all the water was either locked up in magma or floating in the atmosphere as steam. In fact, when the Earth was first coalescing from debris in the solar system, compounds of varying densities were mixed together. As the planet grew larger, it underwent a period of stratification during which gravity pulled the densest materials toward the center. Stratification led to off-gassing, where the least dense materialsthe gaseswere spun out into the atmosphere. The released gases consisted primarily of water, but also included small proportions of carbon dioxide, hydrogen, sulfur dioxide, and methane. Only when this planet finally began to cool off was the water vapor able to obtain a liquid form and begin pooling in low spots. It is likely that by about 4 billion years ago, the ocean was essentially fully formed, and it probably covered a similar percentage of the globes surface as it does now (though it was likely shallower then).
Of course, when we think of the ocean, we think of saltwater. However, the salt in seawater is not just regular table salt, which is comprised of sodium and chloride. Instead, seawater has a whole slew of constituent minerals dissolved in its solution. It turns out that there are at least 56 different elements detectable in seawater. Many, but not all, of these elements contribute to the waters overall salinity. A salt is merely defined as the product of the reaction of an acid and a base, so there are many possible minerals that fit this definition. Regarding salinity measurements, it is the relative abundance of inorganic ions that affects the overall value. Moreover, only a few major elements, such as sodium, chlorine, potassium, calcium, magnesium, strontium, and a couple others, are found in concentrations high enough to greatly impact salinity.
Now that we have some idea of what salt actually is, we still need to know from whence it came. Actually, the answer is pretty simpleit dissolved from rocks and atmospheric gases. The processes that erode away mountains and carve the worlds spectacular canyons also dissolve salts from the rocks, and washes them out into the ocean. Though we think of freshwater as being salt free, it actually contains a small percentage of salt as well. In most cases, freshwater is about ten times less salty than seawater. As the water falls in the form of rain, and migrates towards the sea, it picks up small amounts of salt from the rocks and sediment around which it flows. The atmosphere also supplies salt to the ocean because the interface between the two is very extensive, allowing gas to dissolve in the water. In a very real way, the atmosphere and ocean can be considered two parts of the same whole, so what is in one is also in the other. In general, the salt composition is dictated by both the relative abundance of each element, and its solubility in water. In the ocean, the biological and chemical processes that export salt exist in equilibrium with the inputs, and so salinity stays fairly stable.
Measuring Salinity
When we measure the salinity of seawater, we can use one of several methods. Different methods measure different properties, and so express values differently. The most accurate, by far, is that which uses a salinometer. Such devices are able to measure the electrical conductivity in a water sample, and compare the value to a known standard. They produce results in units of parts of inorganic ions per thousand (ppT), which is the most direct measurement of salinity. Natural seawater has an average value of 35 ppT, though it has become increasingly common for people to drop the units all together. Therefore, saying that the salinity is 35 implies that the salt content is equivalent to 3.5%. Though they are highly accurate, salinometers are very expensive. More commonly, salinity is measured as a function of the water samples density. Hydrometers and refractometers both measure density, and express it in terms of specific gravity (SG). Hydrometers come in several varieties, but all use the same principle to do their jobs. That is, hydrometers measure the buoyancy of an object of specific density that is floated in a water sample. Many hydrometers are very inaccurate, while others only function correctly when used with water of a precise temperature. Refractometers, on the other hand, bend light through a prism to give a measurement of density. Most refractometers on the market today automatically adjust for temperature, and can easily be calibrated at home (many also measure salinity simultaneously). They are by far the best option available to home aquarists. Seawater, as previously mentioned, has a SG of 1.024, which is equivalent to a salinity of 35. It should be noted, however, that specific gravity is not a measurement of salinity. Rather, it is a relative comparison of the densities of pure water and impure (read salt) water. In other words, seawater is 1.024 times denser than pure fresh water.
Biological Impact of Salt
As I alluded to earlier in this article, saltwater aquaria are generally maintained between 1.021 and 1.025 SG. You may wonder why we would not try to keep them at a rigid 1.024 to match natural seawater. The answer is simply that the majority of our livestock survive well anywhere within this continuum, provided that the salinity remains stable. Stability is more critical than keeping the perfect percentage of salt.
One way to categorize marine animals is by how they deal with salt regulation. Salt, like any impurity in solution, will move from areas of high concentration to areas of low concentration. Scientists refer to the differential as a concentration gradient, and the concept is very important in biological systems. When a semi-permeable or permeable membrane divides the concentration, ions move across spontaneously in a process called osmosis. Those organisms that passively allow salt to enter and leave their bodies are called osmoconformers, which means that they do not exert osmotic control, and internal salt levels basically match those of the surrounding environment. Invertebrate animals such as snails, corals, and crustaceans, along with bacteria and algae, are all osmoconformers. As such, it is necessary to keep a tank with these animals within the aforementioned salinity ranges. Fish and other vertebrates are called osmoregulators, meaning that they actively control internal salt levels through metabolic activities. They must constantly release salt by urinating, because their bodies are designed to function at a level which is hyposaline (less salty) than the surrounding hypersaline (saltier) water. Osmoregulators must expend metabolic energy to counteract the osmotic pressure that is constantly trying to suck water out, and force ions in.
The benefit of fish being osmoregulators is that they can survive for weeks in water that is far less salty than seawater. In fact, lowered salinity (around 1.010 SG) is therapeutic for fish because it reduces the osmotic pressure with which the animals must contend. When saltwater fish are placed in hyposaline water they waste less energy extruding salt, which leaves more energy to be put into immune defense. Accordingly, hyposalinity treatment is one viable option available for aquarists seeking to rehabilitate sick or injured fish. Another added benefit to hyposalinity is that it kills invertebrates, thus many parasites and pathogens are unable to survive extended periods in dilute water (corals, beneficial bacteria, and algae will also be killedhyposalinity treatment must only be performed in a quarantine tank). Interestingly, fish can be moved directly from normal salinity to hyposalinity, with no ill effects. However, the reverse is not true. Fish cannot be returned to normal salinity immediately. They must be slowly brought back to normal salinity, with increases of no more than .002 SG per day.
In Closing
Controlling salinity in an aquarium is clearly a paramount husbandry issue. Fortunately, doing so is pretty simple. Using a refractometer is one of the best ways to maintain salinity accurately and precisely. They also do their jobs quickly and efficiently, saving you time and using less water. Once the proper salinity is established, it tends to change very slowly. Good husbandry practices will offset the loss of freshwater through evaporation, as well as the loss of salt via protein skimming and salt creep. As long as you check salinity regularly, perform top-offs with buffered freshwater, and do water changes often, you should never have a problem.
Works Cited:
Castro, P. and M.E. Huber. Marine Biology, 5th Edition. McGraw Hill: Boston. 2005.
Brightwell, C.R. Marine Chemistry; A Complete Guide to Water Chemistry in Marine Aquariums. TFH Publications: Neptune City. 2007.
Thurman, H.V. and A.P. Trujillo. Introductory Oceanography, 10th Edition. Pearson Prentice Hall: Upper Saddle River. 2004.
Goemans, B. and L. Ichinotsubo. The Marine Fish Health and Feeding Handbook; The Essential Guide to Keeping Saltwater Species Alive and Thriving. TFH Publications: Neptune City. 2008.
Silberberg, M.S. Chemistry; the Molecular Nature of Matter and Change, 3rd Edition. McGraw Hill: Boston. 2003.